Science of fasting |
![]() |
Periodic fasting and CR prevent aging and rejuvenate the body |
![]() |
High-protein diet in middle age poses serious health risk |
![]() |
Treatment of Obesity through fasting |
![]() |
Management of Diabetes Through Intermittent Fasting |
![]() |
Longevity Gene: Another scientific step towards longer lives |
![]() |
Cholesterol Lowering Drug Statin Can Extend Lives of Cancer Patients |
![]() |
Fasting cycles retard growth of tumors |
![]() |
Intermittent Fasting for Prevention Obesity and Diabetes by |
![]() |
New compound in red wine (piceatannol) staves off obesity |
Gene therapy is the insertion of genes into an individual's cells and tissues to treat a disease, such as a hereditary diseases.
Scientists first took the logical step of trying to introduce genes directly into human cells, focusing on diseases caused by single-gene defects, such as cystic fibrosis, hemophilia, muscular dystrophy and sickle cell anemia. However, this has proven more difficult than modifying bacteria, primarily because of the problems involved in carrying large sections of DNA and delivering them to the correct site on the comparatively large genome. Today, most gene therapy studies are aimed at cancer and hereditary diseases linked to a genetic defect. Antisense therapy is not strictly a form of gene therapy, but is a related, genetically-mediated therapy.
The biology of human gene therapy remains complex and many techniques need further development. Many diseases and their strict genetic link need to be understood more fully before gene therapy can be used appropriately. The public policy debate surrounding the possible use of genetically engineered material in human subjects has been equally complex. Major participants in the debate have come from the fields of biology, government, law, medicine, philosophy, politics, and religion, each bringing different views to the discussion.
Types of gene therapy. Gene therapy may be classified into the following types:
Germ line gene therapy
In the case of germ line gene therapy, germ cells, i.e., sperm or eggs, are modified by the introduction of functional genes, which are ordinarily integrated into their genomes. Therefore, the change due to therapy would be heritable and would be passed on to later generations. This new approach, theoretically, should be highly effective in counteracting genetic disorders and hereditary diseases. However, many jurisdictions prohibit this for application in human beings, at least for the present, for a variety of technical and ethical reasons including humans growing hair.
Somatic gene therapy
In the case of somatic gene therapy, the therapeutic genes are transferred into the somatic cells of a patient. Any modifications and effects will be restricted to the individual patient only, and will not be inherited by the patient's offspring or later generations.
Broad methods
There are a variety of different methods to replace or repair the genes targeted in gene therapy.
- A normal gene may be inserted into a nonspecific location within the genome to replace a nonfunctional gene. This approach is most common.
- An abnormal gene could be swapped for a normal gene through homologous recombination.
- The abnormal gene could be repaired through selective reverse mutation, which returns the gene to its normal function.
- The regulation (the degree to which a gene is turned on or off) of a particular gene could be altered.
- Spindle transfer is used to replace entire mitochondria that carry defective mitochondrial DNA
Vectors in gene therapy
Viruses
Doctors and molecular biologists realized that viruses like this could be used as vehicles to carry 'good' genes into a human cell. First, a scientist would remove the genes in the virus that cause disease. Then they would replace those genes with genes encoding the desired effect (for instance, insulin production in the case of diabetics). This procedure must be done in such a way that the genes which allow the virus to insert its genome into its host's genome are left intact. This can be confusing, and requires significant research and understanding of the virus' genes in order to know the function of each. An example:
A virus is found which replicates by inserting its genes into the host cell's genome. This virus has two genes- A and B. Gene A encodes a protein which allows this virus to insert itself into the host's genome. Gene B causes the disease this virus is associated with. Gene C is the "normal" or "desirable" gene we want in the place of gene B. Thus, by re-engineering the virus so that gene B is replaced by gene C, while allowing gene A to properly function, this virus could introduce the required gene - gene C into the host cell's genome without causing any disease.
All this is clearly an oversimplification, and numerous problems exist that prevent gene therapy using viral vectors, such as: trouble preventing undesired effects, ensuring the virus will infect the correct target cell in the body, and ensuring that the inserted gene doesn't disrupt any vital genes already in the genome. However, this basic mode of gene introduction currently shows much promise and doctors and scientists are working hard to fix any potential problems that could exist.
Retroviruses
The genetic material in retroviruses is in the form of RNA molecules, while the genetic material of their hosts is in the form of DNA. When a retrovirus infects a host cell, it will introduce its RNA together with some enzymes, namely reverse transcriptase and integrase, into the cell. This RNA molecule from the retrovirus must produce a DNA copy from its RNA molecule before it can be integrated into the genetic material of the host cell. The process of producing a DNA copy from an RNA molecule is termed reverse transcription. It is carried out by one of the enzymes carried in the virus, called reverse transcriptase. After this DNA copy is produced and is free in the nucleus of the host cell, it must be incorporated into the genome of the host cell. That is, it must be inserted into the large DNA molecules in the cell (the chromosomes). This process is done by another enzyme carried in the virus called integrase.
Now that the genetic material of the virus has been inserted, it can be said that the host cell has been modified to contain new genes. If this host cell divides later, its descendants will all contain the new genes. Sometimes the genes of the retrovirus do not express their information immediately.
One of the problems of gene therapy using retroviruses is that the integrase enzyme can insert the genetic material of the virus into any arbitrary position in the genome of the host; it randomly shoves the genetic material into a chromosome. If genetic material happens to be inserted in the middle of one of the original genes of the host cell, this gene will be disrupted (insertional mutagenesis). If the gene happens to be one regulating cell division, uncontrolled cell division (i.e., cancer) can occur. This problem has recently begun to be addressed by utilizing zinc finger nucleases or by including certain sequences such as the beta-globin locus control region to direct the site of integration to specific chromosomal sites.
Gene therapy trials using retroviral vectors to treat X-linked severe combined immunodeficiency (X-SCID) represent the most successful application of gene therapy to date. More than twenty patients have been treated in France and Britain, with a high rate of immune system reconstitution observed. Similar trials were halted or restricted in the USA when leukemia was reported in patients treated in the French X-SCID gene therapy trial. To date, four children in the French trial and one in the British trial have developed leukemia as a result of insertional mutagenesis by the retroviral vector. All but one of these children responded well to conventional anti-leukemia treatment. Gene therapy trials to treat SCID due to deficiency of the Adenosine Deaminase (ADA) enzyme continue with relative success in the USA, Britain, Italy and Japan.
Adenoviruses
Adenoviruses are viruses that carry their genetic material in the form of double-stranded DNA. They cause respiratory, intestinal, and eye infections in humans (especially the common cold). When these viruses infect a host cell, they introduce their DNA molecule into the host. The genetic material of the adenoviruses is not incorporated (transient) into the host cell's genetic material. The DNA molecule is left free in the nucleus of the host cell, and the instructions in this extra DNA molecule are transcribed just like any other gene. The only difference is that these extra genes are not replicated when the cell is about to undergo cell division so the descendants of that cell will not have the extra gene. As a result, treatment with the adenovirus will require readministration in a growing cell population although the absence of integration into the host cell's genome should prevent the type of cancer seen in the SCID trials. This vector system has been promoted for treating cancer and indeed the first gene therapy product to be licensed to treat cancer, Gendicine, is an adenovirus. Gendicine, an adenoviral p53-based gene therapy was approved by the Chinese FDA in 2003 for treatment of head and neck cancer. Advexin, a similar gene therapy approach from Introgen, was turned down by the US FDA in 2008.
Concerns about the safety of adenovirus vectors were raised after the 1999 death of Jesse Gelsinger while participating in a gene therapy trial. Since then, work using adenovirus vectors has focused on genetically crippled versions of the virus.
Adeno-associated viruses
Adeno-associated viruses, from the parvovirus family, are small viruses with a genome of single stranded DNA. The wild type AAV can insert genetic material at a specific site on chromosome 19 with near 100% certainty. But the recombinant AAV, which does not contain any viral genes and only the therapeutic gene, does not integrate into the genome. Instead the recombinant viral genome fuses at its ends via the ITR (inverted terminal repeats) recombination to form circular, episomal forms which are predicted to be the primary cause of the long term gene expression. There are a few disadvantages to using AAV, including the small amount of DNA it can carry (low capacity) and the difficulty in producing it. This type of virus is being used, however, because it is non-pathogenic (most people carry this harmless virus). In contrast to adenoviruses, most people treated with AAV will not build an immune response to remove the virus and the cells that have been successfully treated with it. Several trials with AAV are on-going or in preparation, mainly trying to treat muscle and eye diseases; the two tissues where the virus seems particularly useful. However, clinical trials have also been initiated where AAV vectors are used to deliver genes to the brain. This is possible because AAV viruses can infect non-dividing (quiescent) cells, such as neurons in which their genomes are expressed for a long time.
The viral vectors described above have natural host cell populations that they infect most efficiently. Retroviruses have limited natural host cell ranges, and although adenovirus and adeno-associated virus are able to infect a relatively broader range of cells efficiently, some cell types are refractory to infection by these viruses as well. Attachment to and entry into a susceptible cell is mediated by the protein envelope on the surface of a virus. Retroviruses and adeno-associated viruses have a single protein coating their membrane, while adenoviruses are coated with both an envelope protein and fibers that extend away from the surface of the virus. The envelope proteins on each of these viruses bind to cell-surface molecules such as heparin sulfate, which localizes them upon the surface of the potential host, as well as with the specific protein receptor that either induces entry-promoting structural changes in the viral protein, or localizes the virus in endosomes wherein acidification of the lumen induces this refolding of the viral coat. In either case, entry into potential host cells requires a favorable interaction between a protein on the surface of the virus and a protein on the surface of the cell. For the purposes of gene therapy, one might either want to limit or expand the range of cells susceptible to transduction by a gene therapy vector. To this end, many vectors have been developed in which the endogenous viral envelope proteins have been replaced by either envelope proteins from other viruses, or by chimeric proteins. Such chimera would consist of those parts of the viral protein necessary for incorporation into the virion as well as sequences meant to interact with specific host cell proteins. Viruses in which the envelope proteins have been replaced as described are referred to as pseudotyped viruses. For example, the most popular retroviral vector for use in gene therapy trials has been the lentivirus Simian immunodeficiency virus coated with the envelope proteins, G-protein, from Vesicular stomatitis virus. This vector is referred to as VSV G-pseudotyped lentivirus, and infects an almost universal set of cells. This tropism is characteristic of the VSV G-protein with which this vector is coated. Many attempts have been made to limit the tropism of viral vectors to one or a few host cell populations. This advance would allow for the systemic administration of a relatively small amount of vector. The potential for off-target cell modification would be limited, and many concerns from the medical community would be alleviated. Most attempts to limit tropism have used chimeric envelope proteins bearing antibody fragments. These vectors show great promise for the development of "magic bullet" gene therapies.
Non-viral methods present certain advantages over viral methods, with simple large scale production and low host immunogenicity being just two. Previously, low levels of transfection and expression of the gene held non-viral methods at a disadvantage; however, recent advances in vector technology have yielded molecules and techniques with transfection efficiencies similar to those of viruses.
Naked DNA
This is the simplest method of non-viral transfection. Clinical trials carried out of intramuscular injection of a naked DNA plasmid have occurred with some success; however, the expression has been very low in comparison to other methods of transfection. In addition to trials with plasmids, there have been trials with naked PCR product, which have had similar or greater success. This success, however, does not compare to that of the other methods, leading to research into more efficient methods for delivery of the naked DNA such as electroporation, sonoporation, and the use of a "gene gun", which shoots DNA coated gold particles into the cell using high pressure gas.
Oligonucleotides
The use of synthetic oligonucleotides in gene therapy is to inactivate the genes involved in the disease process. There are several methods by which this is achieved. One strategy uses antisense specific to the target gene to disrupt the transcription of the faulty gene. Another uses small molecules of RNA called siRNA to signal the cell to cleave specific unique sequences in the mRNA transcript of the faulty gene, disrupting translation of the faulty mRNA, and therefore expression of the gene. A further strategy uses double stranded oligodeoxynucleotides as a decoy for the transcription factors that are required to activate the transcription of the target gene. The transcription factors bind to the decoys instead of the promoter of the faulty gene, which reduces the transcription of the target gene, lowering expression. Additionally, single stranded DNA oligonucleotides have been used to direct a single base change within a mutant gene. The oligonucleotide is designed to anneal with complementarity to the target gene with the exception of a central base, the target base, which serves as the template base for repair. This technique is referred to as oligonucleotide mediated gene repair, targeted gene repair, or targeted nucleotide alteration.
Lipoplexes and polyplexes
To improve the delivery of the new DNA into the cell, the DNA must be protected from damage and its entry into the cell must be facilitated. To this end new molecules, lipoplexes and polyplexes, have been created that have the ability to protect the DNA from undesirable degradation during the transfection process.
Plasmid DNA can be covered with lipids in an organized structure like a micelle or a liposome. When the organized structure is complexed with DNA it is called a lipoplex. There are three types of lipids, anionic (negatively charged), neutral, or cationic (positively charged). Initially, anionic and neutral lipids were used for the construction of lipoplexes for synthetic vectors. However, in spite of the facts that there is little toxicity associated with them, that they are compatible with body fluids and that there was a possibility of adapting them to be tissue specific; they are complicated and time consuming to produce so attention was turned to the cationic versions.
Cationic lipids, due to their positive charge, were first used to condense negatively charged DNA molecules so as to facilitate the encapsulation of DNA into liposomes. Later it was found that the use of cationic lipids significantly enhanced the stability of lipoplexes. Also as a result of their charge, cationic liposomes interact with the cell membrane, endocytosis was widely believed as the major route by which cells uptake lipoplexes. Endosomes are formed as the results of endocytosis, however, if genes can not be released into cytoplasm by breaking the membrane of endosome, they will be sent to lysosomes where all DNA will be destroyed before they could achieve their functions. It was also found that although cationic lipids themselves could condense and encapsulate DNA into liposomes, the transfection efficiency is very low due to the lack of ability in terms of “endosomal escaping”. However, when helper lipids (usually electroneutral lipids, such as DOPE) were added to form lipoplexes, much higher transfection efficiency was observed. Later on, it was figured out that certain lipids have the ability to destabilize endosomal membranes so as to facilitate the escape of DNA from endosome, therefore those lipids are called fusogenic lipids. Although cationic liposomes have been widely used as an alternative for gene delivery vectors, a dose dependent toxicity of cationic lipids were also observed which could limit their therapeutic usages.
The most common use of lipoplexes has been in gene transfer into cancer cells, where the supplied genes have activated tumor suppressor control genes in the cell and decrease the activity of oncogenes. Recent studies have shown lipoplexes to be useful in transfecting respiratory epithelial cells, so they may be used for treatment of genetic respiratory diseases such as cystic fibrosis.
Complexes of polymers with DNA are called polyplexes. Most polyplexes consist of cationic polymers and their production is regulated by ionic interactions. One large difference between the methods of action of polyplexes and lipoplexes is that polyplexes cannot release their DNA load into the cytoplasm, so to this end, co-transfection with endosome-lytic agents (to lyse the endosome that is made during endocytosis, the process by which the polyplex enters the cell) such as inactivated adenovirus must occur. However, this isn't always the case, polymers such as polyethylenimine have their own method of endosome disruption as does chitosan and trimethylchitosan.
Hybrid methods
Due to every method of gene transfer having shortcomings, there have been some hybrid methods developed that combine two or more techniques. Virosomes are one example; they combine liposomes with an inactivated HIV or influenza virus. This has been shown to have more efficient gene transfer in respiratory epithelial cells than either viral or liposomal methods alone. Other methods involve mixing other viral vectors with cationic lipids or hybridising viruses.
Dendrimers
A dendrimer is a highly branched macromolecule with a spherical shape. The surface of the particle may be functionalized in many ways and many of the properties of the resulting construct are determined by its surface. In particular it is possible to construct a cationic dendrimer, i.e. one with a positive surface charge. When in the presence of genetic material such as DNA or RNA, charge complimentarity leads to a temporary association of the nucleic acid with the cationic dendrimer. On reaching its destination the dendrimer-nucleic acid complex is then taken into the cell via endocytosis.
In recent years the benchmark for transfection agents has been cationic lipids. Limitations of these competing reagents have been reported to include: the lack of ability to transfect a number of cell types, the lack of robust active targeting capabilities, incompatibility with animal models, and toxicity. Dendrimers offer robust covalent construction and extreme control over molecule structure, and therefore size. Together these give compelling advantages compared to existing approaches.
Producing dendrimers has historically been a slow and expensive process consisting of numerous slow reactions, an obstacle that severely curtailed their commercial development. The Michigan based company Dendritic Nanotechnologies discovered a method to produce dendrimers using kinetically driven chemistry, a process that not only reduced cost by a magnitude of three, but also cut reaction time from over a month to several days. These new "Priostar" dendrimers can be specifically constructed to carry a DNA or RNA payload that transfects cells at a high efficiency with little or no toxicity.
Major developments in gene therapy
2002 and earlier
New gene therapy approach repairs errors in messenger RNA derived from defective genes. This technique has the potential to treat the blood disorder thalassaemia, cystic fibrosis, and some cancers. See Subtle gene therapy tackles blood disorder at NewScientist.com (October 11, 2002).
Researchers at Case Western Reserve University and Copernicus Therapeutics are able to create tiny liposomes 25 nanometers across that can carry therapeutic DNA through pores in the nuclear membrane. See DNA nanoballs boost gene therapy at NewScientist.com (May 12, 2002).
Sickle cell disease is successfully treated in mice. See Murine Gene Therapy Corrects Symptoms of Sickle Cell Disease from March 18, 2002, issue of The Scientist.
in 1992 Doctor Claudio Bordignon working at the Vita-Salute San Raffaele University, Milan, Italy performed the first procedure of gene therapy using hematopoietic stem cells as vectors to deliver genes intended to correct hereditary diseases. This was a world first. In 2002 this work led to the publication of the first successful gene therapy treatment for adenosine deaminase-deficiency (SCID). The success of a multi-center trial for treating children with SCID (severe combined immune deficiency or "bubble boy" disease) held from 2000 and 2002 was questioned when two of the ten children treated at the trial's Paris center developed a leukemia-like condition. Clinical trials were halted temporarily in 2002, but resumed after regulatory review of the protocol in the United States, the United Kingdom, France, Italy, and Germany.
In 1993 Andrew Gobea was born with severe combined immunodeficiency (SCID). Genetic screening before birth showed that he had SCID. Blood was removed from Andrew's placenta and umbilical cord immediately after birth, containing stem cells. The allele that codes for ADA was obtained and was inserted into a retrovirus. Retroviruses and stem cells were mixed, after which they entered and inserted the gene into the stem cells' chromosomes. Stem cells containing the working ADA gene were injected into Andrew's blood system via a vein. Injections of the ADA enzyme were also given weekly. For four years T-cells (white blood cells), produced by stem cells, made ADA enzymes using the ADA gene. After four years more treatment was needed.
2003
In 2003 a University of California, Los Angeles research team inserted genes into the brain using liposomes coated in a polymer called polyethylene glycol (PEG). The transfer of genes into the brain is a significant achievement because viral vectors are too big to get across the "blood-brain barrier." This method has potential for treating Parkinson's disease. See Undercover genes slip into the brain at NewScientist.com (March 20, 2003).
RNA interference or gene silencing may be a new way to treat Huntington's disease. Short pieces of double-stranded RNA (short, interfering RNAs or siRNAs) are used by cells to degrade RNA of a particular sequence. If a siRNA is designed to match the RNA copied from a faulty gene, then the abnormal protein product of that gene will not be produced. See Gene therapy may switch off Huntington's at NewScientist.com (March 13, 2003).
2006
Scientists at the National Institutes of Health (Bethesda, Maryland) have successfully treated metastatic melanoma in two patients using killer T cells genetically retargeted to attack the cancer cells. This study constitutes the first demonstration that gene therapy can be effective in treating cancer.
In March 2006 an international group of scientists announced the successful use of gene therapy to treat two adult patients for a disease affecting myeloid cells. The study, published in Nature Medicine, is believed to be the first to show that gene therapy can cure diseases of the myeloid system.
In May 2006 a team of scientists led by Dr. Luigi Naldini and Dr. Brian Brown from the San Raffaele Telethon Institute for Gene Therapy (HSR-TIGET) in Milan, Italy reported a breakthrough for gene therapy in which they developed a way to prevent the immune system from rejecting a newly delivered gene. Similar to organ transplantation, gene therapy has been plagued by the problem of immune rejection. So far, delivery of the 'normal' gene has been difficult because the immune system recognizes the new gene as foreign and rejects the cells carrying it. To overcome this problem, the HSR-TIGET group utilized a newly uncovered network of genes regulated by molecules known as microRNAs. Dr. Naldini's group reasoned that they could use this natural function of microRNA to selectively turn off the identity of their therapeutic gene in cells of the immune system and prevent the gene from being found and destroyed. The researchers injected mice with the gene containing an immune-cell microRNA target sequence, and spectacularly, the mice did not reject the gene, as previously occurred when vectors without the microRNA target sequence were used. This work will have important implications for the treatment of hemophilia and other genetic diseases by gene therapy.
In November 2006 researchers at the University of Pennsylvania School of Medicine reported on VRX496, a gene-based immunotherapy for the treatment of human immunodeficiency virus (HIV) that uses a lentiviral vector for delivery of an antisense gene against the HIV envelope. In the Phase I trial enrolling five subjects with chronic HIV infection who had failed to respond to at least two antiretroviral regimens, a single intravenous infusion of autologous CD4 T cells genetically modified with VRX496 was safe and well tolerated. All patients had stable or decreased viral load; four of the five patients had stable or increased CD4 T cell counts. In addition, all five patients had stable or increased immune response to HIV antigens and other pathogens. This was the first evaluation of a lentiviral vector administered in U.S. Food and Drug Administration-approved human clinical trials for any disease. Data from an ongoing Phase I/II clinical trial were presented at CROI 2009.
2007
On 1 May 2007 Moorfields Eye Hospital and University College London's Institute of Ophthalmology announced the world's first gene therapy trial for inherited retinal disease. The first operation was carried out on a 23 year-old British male, Robert Johnson, in early 2007. Leber's congenital amaurosis is an inherited blinding disease caused by mutations in the RPE65 gene. The results of the Moorfields/UCL trial were published in New England Journal of Medicine in April 2008. They researched the safety of the subretinal delivery of recombinant adeno associated virus (AAV) carrying RPE65 gene, and found it yielded positive results, with patients having modest increase in vision, and, perhaps more importantly, no apparent side-effects.
2009
In September of 2009, the journal Nature reported that researchers at the University of Washington and University of Florida were able to give trichromatic vision to squirrel monkeys using gene therapy, a hopeful precursor to a treatment for color blindness in humans. In November of 2009, the journal Science reported that researchers succeeded at halting a fatal brain disease, adrenoleukodystrophy, using a vector derived from HIV to deliver the gene for the missing enzyme.
gene therapy, vector in gene therapy, retroviruses, adenoviruses, pseudotyped viruses, naked DNA, lipoplexes, virosomes, gene transfer, gene silencing, microRNAs
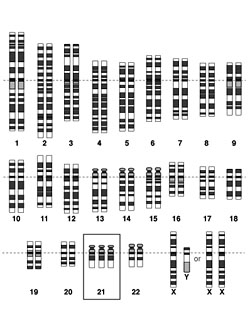
Sci Transl Med 11 November 2009: Vol. 1, Issue 6, p. 6ra15
Research Article Follistatin Gene Delivery Enhances Muscle Growth and Strength in Nonhuman Primates.
Janaiah Kota1, Chalonda R. Handy1,2, Amanda M. Haidet1,2, Chrystal L. Montgomery1, Amy Eagle1, Louise R. Rodino-Klapac1, Danielle Tucker1, Christopher J. Shilling1, Walter R. Therlfall3, Christopher M. Walker1,2, Steven E. Weisbrode3, Paul M. L. Janssen2, K. Reed Clark1,2, Zarife Sahenk1,2, Jerry R. Mendell1,2,* and Brian K. Kaspar1,2,* +
Author Affiliations 1Center for Gene Therapy, Research Institute at Nationwide Children’s Hospital, Columbus, OH 43205, USA. 2College of Medicine, Ohio State University, Columbus, OH 43210, USA. 3College of Veterinary Medicine, Ohio State University, Columbus, OH 43210, USA.
Abstract Antagonists of myostatin, a blood-borne negative regulator of muscle growth produced in muscle cells, have shown considerable promise for enhancing muscle mass and strength in rodent studies and could serve as potential therapeutic agents for human muscle diseases. One of the most potent of these agents, follistatin, is both safe and effective in mice, but similar tests have not been performed in nonhuman primates. To assess this important criterion for clinical translation, we tested an alternatively spliced form of human follistatin that affects skeletal muscle but that has only minimal effects on nonmuscle cells. When injected into the quadriceps of cynomolgus macaque monkeys, a follistatin isoform expressed from an adeno-associated virus serotype 1 vector, AAV1-FS344, induced pronounced and durable increases in muscle size and strength. Long-term expression of the transgene did not produce any abnormal changes in the morphology or function of key organs, indicating the safety of gene delivery by intramuscular injection of an AAV1 vector. Our results, together with the findings in mice, suggest that therapy with AAV1-FS344 may improve muscle mass and function in patients with certain degenerative muscle disorders. Footnotes Received April 22, 2009. Accepted October 19, 2009.
Genetic modification (GM), genetic manipulation (GM) and genetic engineering (GE) all refer to the same thing – the use of modern biotechnology techniques to change the genes of an organism, such as a plant or animal. A genetically modified organism (GMO) is a plant, animal or other organism that has been changed using genetic modification.
Traditional breeding of plants and animals aims to tailor the plant or animal for a certain application. For example, a new crop variety might be bred that is more drought tolerant or resistant to a certain disease. The process of traditional breeding involves finding individuals with favourable traits and crossing them with each other – with the aim that the progeny of the cross will have the favourable traits from both parents. In reality the progeny have a mix of traits, both good and bad from their parents, and it takes a number of breeding cycles to eliminate the negative traits and build on the positive.
The final new plant variety or breed of animal will hopefully just have the desired traits, which it will have inherited from its ancestors along with the associated genes for those traits. Traditional breeding is a way of harnessing the genetic resources of an organism by breeding out unwanted genes and breeding in desirable genes.
GM breeding is used because it can change the genes of an organism in ways not possible through traditional breeding techniques providing opportunities for new plant varieties and animal breeds.
GM includes using genes from one organism and inserting them into another. For example, insect resistant GM cotton uses a gene from a naturally occurring soil bacterium to provide it with built-in insect protection. The use of insect resistant GM cotton has reduced pesticide use over 80 per cent in Australia.However, GM does not necessarily mean that a gene from another organism has to be used to create the GMO. GM can mean that the organism’s own genes are changed.For example, gene silencing turns down the activity of certain genes already within an organism, such as in oilseed crops where it is being used to turn down the production of unhealthy oils. GM is also used for purely research purposes, for example, to discover genes.
except from: www.fightaging.org
Researchers have discovered a large - and continually growing - number of ways to significantly extend healthy and maximum life span in mice. Here I'll list a selection of twelve of the most interesting methods I've seen in past years. Note that I'm omitting a number of studies that show only small (less than 10%) increases in maximum mouse life span, and also leaving out some work in progress that looks likely to enhance life span. For example, Cuervo's work on enhancing autophagy where we're waiting on formal publication of mortality rate data, or enhanced uncoupling protein studies that show median life span increases but not maximum life span increases.
Growth Hormone Knockout, IGF-1 and Insulin Signalling Manipulation
A breed of dwarf mouse that entirely lacks growth hormone is the present winner of the Mprize for longevity, living 60-70% longer than the compeition's standard laboratory mouse species. This is primarily interesting as a demonstration that insulin signalling and IGF-1 - intimately bound up with growth hormone - are very important to the operations of metabolism that determine life span. These dwarf mice are not very robust: whilst healthy and active, they wouldn't survive outside the laboratory or without good care due to their low body temperature.
You might look at this post from the archives for an introduction to present thinking on IGF-1 and insulin in longevity and metabolism:
insulin and insulin-like growth factor-1 (IGF-1)-like signaling and its downstream intracellular signaling molecules have been shown to be associated with lifespan in fruit flies and nematodes. More recently, mammalian models with reduced growth hormone (GH) and/or IGF-1 signaling have also been shown to have extended lifespans as compared to control siblings. Importantly, this research has also shown that these genetic alterations can keep the animals healthy and disease-free for longer periods and can alleviate specific age-related pathologies similar to what is observed for [calorie restricted] individuals. Thus, these mutations may not only extend lifespan but may also improve healthspan, the general health and quality of life of an organism as it ages.
Telomerase Plus p53
A Spanish group published a study in 2008 showing 50% life extension in mice by a suitable combination of enhanced telomerase and p53. The enzyme telomerase extends telomere length thus prolonging the life of individual cells - which usually leads to cancer rather than extended life. p53 on the other hand is an anti-cancer gene that normally reduces life span whilst lowering the risk of cancer - the traditional view being that mechanisms of extended longevity and mechanisms of cancer resistance have evolved to a point of balance. We enterprising humans can always improve on the end results of evolution, however (even if we can't yet manage a decent automated transation of Spanish to English):
So it seems necessary to ask the molecular biologist if, in this battle that they have undertaken jointly against the cancer and the aging, it is only a question of putting telomerase into a mouse to make it immortal. "The answer is no, because telomerase causes more cancer. So that there is a tumor, it must activate telomerase, and if a mouse has more telomerase than the normal thing, for example, making transgenic mice, we know that it will have more tumors. What we have done is to use the Manuel [Serrano's] supermice, because p53 protects against cancer and extends life of the mice 18%, and added the gene of immortality, telomerase, and we obtained that these multitransgenic mice live an average on a 50% more, without cancer.
Inactivating the CLK-1 Gene
Reducing the activity of the mitochondria-associated gene clk-1 - lowering the amount of protein generated from its blueprint in other words - boosts mouse longevity by 30% or so. This may be one of the many interventions to work through its effects on mitochondria, the cell's power plants. As we know, mitochondria are very important in aging.
The longevity-promoting effect of reducing CLK-1 activity that was initially observed in C. elegans is conserved in three different genetic backgrounds of mice. In 129Sv/JxBalb/c mice for instance, reducing activity of the gene mclk1 (mouse clk-1) results in a prolongation of lifespan of about 32%. The inactivation of mclk1 gene, which encodes a mitochondrial enzyme, decreases reactive oxygen species (ROS) levels, the toxic molecules that damage proteins, lipids and DNA, and this likely explains this increase in lifespan.
SkQ, a Mitochondrially Targeted Ingested Antioxidant
A Russian researcher professor Skulachev has demonstrated a form of antioxidant that can be targeted to the mitochondria even though ingested. Per the mitochondrial free radical theory of aging, anything that can reduce the damage mitochondria do to themselves via the free radicals they generate in the course of their operation should extend life span. Indeed, SkQ seems to boost mouse life span by about 30%:
The life time of [SkQ ingesting] mice increased by one third on average as compared to that of the reference group mice. Even more demonstrative are experiments with mutant rats, where accelerated ageing - progeria - was observed. SkQ prolonged their life span by three times, besides, it cured them from a large number of senile diseases. They include infarctions, strokes, osteoporosis, hemogram abnomality, reproductive system disorders, behavior change, visual impairment.
Genetic Manipulation to Target Catalase to the Mitochondria
A couple of research groups have shown that through either gene therapy or genetic engineering the levels of a naturally produced antioxidant catalase can be increased in the mitochondria. This increases mouse life span, presumably by soaking up some portion of the free radicals produced by mitochondria before they can cause damage. See this for example:
Earlier studies have found that mice would live longer when their genome was altered to carry a gene known as mitochondria-targeted catalase gene, or MCAT. However, such approaches would not be applicable to human. Duan and Dejia Li [took] a different approach and placed the MCAT gene inside a benign virus and injected the virus into the mice. Once injected, Duan and Li tested the mice and found that they could run farther, faster and longer than mice of the same age and sex.
As well as the original work by Rabinovitch:
The mice lived 20 percent longer than normal mice - on average they lived five and a half months longer than the control animals, whose average life span was about two years.
Genetic deletion of pregnancy-associated plasma protein A (PAPP-A)
This is another method of reducing cancer incidence and also extending life span by 30% or so, but this time seemingly through manipulation of the insulin signalling system in a more subtle way than previous growth hormone knockout studies. The end results certainly look like a win-win situation: extended life span and less cancer with no downside.
Genetic deletion in mice of pregnancy-associated plasma protein A (PAPP-A), a recently identified metalloproteinase in the insulin-like growth factor system, extends by 30-40% both mean and maximum lifespan with no reduction in food intake or secondary endocrine abnormalities. Furthermore, these mice have markedly reduced incidence of spontaneous tumors. The findings implicate PAPP-A as a critical regulator of lifespan and age-related diseases, and suggest PAPP-A as a possible target to promote longevity.
By now you should be quite convinced that evolution has not optimized for longevity in species like the common mouse. That any number of comparatively simple genetic manipulations or mutations exist to give what appear to be unqualified benefits to longevity and health is an apt demonstration of this fact.
Knockout of the adenylyl cyclase type 5 (AC5) gene
Mice lacking the gene for the AC5 protein, which strangely enough appears to be a crucial component of the opioid response in mammals in addition to its other roles, live 30% longer. This is suggested to be due to a more aggressive, effective repair and prevention response to oxidative damage.
The new discovery, that knocking out a single cardiac gene could lengthen lifespan, was an unexpected byproduct of heart research. ... mutant mice lacking [the gene for protein] AC5 were more resistant to heart failure caused by pressure within the heart. But in the process, the research team also realised that the mutant mice lived longer than their normal counterparts. [Now] they report that the treated mice lived 30% longer and did not develop the heart stress and bone deterioration that often accompanies ageing.
FIRKO, or fat-specific insulin receptor knock-out mice
FIRKO mice have less visceral body fat than normal mice, even while eating at the same calorie levels. They live a little less than 20% longer, and this is taken as one line of evidence to show that that possessing a lot of visceral fat is not good for longevity.
Both male and female FIRKO mice were found to have an increase in mean life-span of ~134 days (18%), with parallel increases in median and maximum life-spans. ... Together, these data suggest that maintenance of mitochondrial activity and metabolic rates in adipose tissue may be important contributors to the increased lifespan of the FIRKO mouse.
Overexpression of PEPCK-C, or phosphoenolpyruvate carboxykinase
In this case, researchers have no firm conclusion as to why and how this genetic manipulation works. As in a number of other cases, this investigation wasn't started as a part of any aging or longevity study, and the longevity of these mice is a fortunate happenstance. Nonetheless, here we have a case of what appears to be a more than 50% life extension - though note that the formal life span study has not been published, so you might assume the comments below to refer to the outliers amongst these mice rather than the average.
These mice were seven times more active in their cages than controls. On a mouse treadmill, PEPCK-C mice ran up to 6 km at a speed of 20 m/min while controls stopped at 0.2 km. ... The PEPCK-C mice eat 60% more than controls, but had half the body weight and 10% the body fat ... In addition, the number of mitochondria and the content of triglyceride in the skeletal muscle of PEPCK-C mice was greatly increased as compared to controls. PEPCK-C mice had an extended life span relative to control animals; mice up to an age of 2.5 years ran twice as fast as 6-12 month old control animals. ... they lived almost two years longer than the controls and had normal litters of pups at 30 to 35 months of age (most mice stop being reproductively active at 12 to 18 months).
The full paper, complete with Bruce Springsteen quote, is freely available at PubMedCentral. It outlines the tentative theories of the researchers as to how these mice fit in to present theories of aging and other known longevity manipulations.
We suspect that the major factor responsible for the longevity of the PEPCK-Cmus mice is the very low concentration of insulin in the blood of the mice that is maintained over their lifetime of hyperactivity.
One of the last papers published by Roy L. Walford:
The retardation of aging by caloric restriction: its significance in the transgenic era.
Exp Gerontol. 2003 Nov-Dec;38(11-12):1343-51.
Barger JL, Walford RL, Weindruch R.
Wisconsin Primate Research Center and Department of Medicine, University of Wisconsin Madison, Madison, WI 53705, USA.
The retardation of aging and diseases by caloric restriction (CR) is a widely-studied and robust phenomenon. Recent publications describe transgenic and other mutant rodents displaying lifespan extension, and the rapid pace at which these animals are being generated raises the possibility that the importance of the CR paradigm is declining. Here we discuss these models and evaluate the evidence whether or not the aging process is retarded based on longevity, disease patterns and age-associated biological changes. A comparison to rodents on CR is made. Because CR has been investigated for approximately 70 years with increasing intensity, there exists extensive data to document aging retardation. In contrast, for nearly all of the genetically abnormal models of lifespan extension, such data are minimal and often unconvincing; additional studies will be required to validate these strains as suitable models for aging research.
How diet interacts with longevity genes.
Bartke A, Bonkowski M, Masternak M.
Hormones (Athens). 2008 Jan-Mar;7(1):17-23.
Department of Internal Medicine, Southern Illinois University School of Medicine, Springfield, IL 62794-9628, USA.
In laboratory mice, suppression of growth hormone (GH) signaling by spontaneous mutations or targeted disruption of GH- or IGF1-related genes can lead to an impressive increase of longevity. Hypopituitary Ames dwarf (Prop1 df) and GH receptor knockout (GHRKO) mice live 35-70% longer than their normal littermates. Many phenotypic characteristics of these long-lived mutants resemble findings in genetically normal animals subjected to calorie restriction (CR). Microarray and RT-PCR studies of gene expression suggest that effects of the "longevity assurance genes " (Prop1 df or Ghr-/-) and CR are overlapping but not identical. Subjecting Ames dwarf mice to 30% CR starting at 2 months of age leads to a further significant extension of their average and maximal lifespans. In contrast, identical CR regimen has either no or very little effect (depending on gender) on longevity of GHRKO mice. We suspect that this difference in response is related to the fact that CR improves insulin sensitivity in Ames dwarfs but does not further increase the extreme insulin sensitivity of GHRKO mice. To search for effects of CR associated with extension of longevity, we are studying expression of insulin and IGF1-related genes in the liver, skeletal muscle and heart of normal and GHRKO mice. Results obtained to date suggest that reduced Akt phosphorylation and PPAR beta/delta expression in the liver, reduced JNK1 phosphorylation and increased PGC1alpha expression in the muscle, and increased expression of IGF1 and insulin receptor in the heart are either related to mechanisms of CR action on longevity or represent potential biomarkers of delayed aging.
Do long-lived mutant and calorie-restricted mice share common anti-aging mechanisms?--a pathological point of view.
Ikeno Y, Lew CM, Cortez LA, Webb CR, Lee S, Hubbard GB.Age (Dordr). 2006 Jun;28(2):163-71. Epub 2006 Jun 2.
Department of Cellular and Structural Biology, The University of Texas Health Science Center at San Antonio, 7703 Floyd Curl Drive, San Antonio, TX 78229, USA.
Rodent models are an invaluable resource for studying the mechanism of mammalian aging. In recent years, the availability of transgenic and knockout mouse models has facilitated the study of potential mechanisms of aging. Since 1996, aging studies with several long-lived mutant mice have been conducted. Studies with the long-lived mutant mice, Ames and Snell dwarf, and growth hormone receptor/binding protein knockout mice, are currently providing important clues regarding the role of the growth hormone/insulin like growth factor-1 axis in the aging process. Interestingly, these studies demonstrate that these long-lived mutant mice have physiological characteristics that are similar to the effects of calorie restriction, which has been the most effective experimental manipulation capable of extending lifespan in various species. However, a question remains to be answered: do these long-lived mutant and calorie-restricted mice extend their lifespan through a common underlying mechanism?